In this example, the species concentrations cj are given in units of mol/m
3, and the rate constants
kj are expressed in 1/day.
The results of the perfectly mixed system, shown in Figure 3, portrait the concentration profiles of aldicarb and its decay by-products and the concentration transients of the three most toxic species — aldicarb, aldicarb sulfoxide, and aldicarb sulfone — as well as their sum (see
Figure 2 for LD
50 values). Only small amounts of aldicarb remain in the pond after 10 days. Considering the summed-up contributions, contamination levels in the water pond remain high even after several months.
The results shown in Figure 3 indicate that the concentration of aldicarb, in a pond treated as a perfectly mixed system, decay to less than 1% the initial concentration after 10 days. Consider this time scale as a reference for a more detailed model.
The spatial-dependent model focuses on the concentration of the highly toxic species aldicarb (ca), aldicarb sulfoxide (
casx), and aldicarb sulfone (
casn). Therefore, you can disregard the mass balances for the hydrogenolysis products (
cao,
csxo, and
csno).
where C denotes specific moisture capacity (m
-1);
Se is the effective saturation of the soil (dimensionless);
S is a storage coefficient (m
-1);
Hp is the pressure head (m), which is proportional to the dependent variable,
p (Pa);
t is time;
K equals the hydraulic conductivity (m/s);
D is the direction (typically, the
z direction) that represents the vertical elevation (m).
Also, the soil permeability κ (SI unit: m
-2) and hydraulic conductivity
K (SI unit: m/s) are related to the fluid viscosity
μ (SI unit: Pa·s) and density
ρ (SI unit: kg/m
3), and to the acceleration of gravity
g (SI unit: m/s
2) by
In this model, the specific moisture capacity Cm and the effective saturation
Se are taken from the van Genuchten retention model (
Ref. 2). For more details see The Richards’ Equation interface in the
Subsurface Flow Module User’s Guide.
The Transport of Diluted Species in Porous Media interface implements the equation above for one or several species. It describes the time rate of change in two terms: c denotes dissolved concentration (mol/m
3) and
cP the mass of adsorbed contaminant per dry unit weight of soil (mg/kg). Further,
θ denotes the volume fraction of fluid (dimensionless), and
ρb is the soil’s bulk density (kg/m
3). Because
ρb amounts to the dried solid mass per bulk volume, the term
ρbcP gives the solute mass attached to the soil as the concentration changes with time.
In these equations, DLGii are the principal components of the water-air dispersion tensor;
DLGij and
DLGji are the cross terms;
α is the dispersivity (m) where the subscripts “1” and “2” denote longitudinal and transverse dispersivity;
Dm and
DG (m
2/d) are molecular diffusion coefficients; and
τL and
τG give the tortuosity factors for water and air, respectively.
The three solutes — aldicarb, aldicarb sulfoxide, and aldicarb sulfone — have different decay rates, RLi, partition coefficients,
kPi, and volatilization constants,
kGi. All the three solutes attach to soil particles, but only aldicarb and aldicarb sulfone volatilize; aldicarb sulfoxide does not.
The following results come from the space- and time-dependent model. Figure 5 shows the fluid flow in soil after 0.3 days (left) and 1 day (right). The plots illustrate the wetting of the soil with time. As indicated by the arrows, the fluid velocities are relatively high beneath the ponded water.
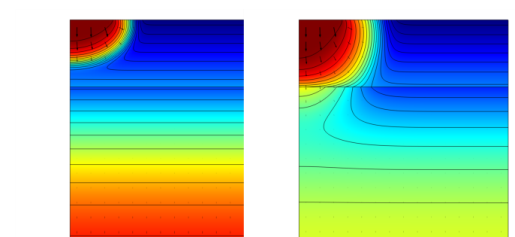
Figure 6 through
Figure 8 show the concentration distributions of aldicarb and the equally toxic aldicarb sulfoxide after 1, 5, and 10 days of infiltration. Consistent with the evolving flow field, the main direction of transport is in the vertical direction.
The distribution of aldicarb has clearly reached steady-state conditions after 10 days, a time frame that was also predicted by the ideal reactor model (see Figure 2). Results also show that the soil contamination is rather local with respect to the aldicarb source. The aldicarb sulfoxide, on the other hand, can be expected to affect a considerably larger soil volume for a significantly longer time.